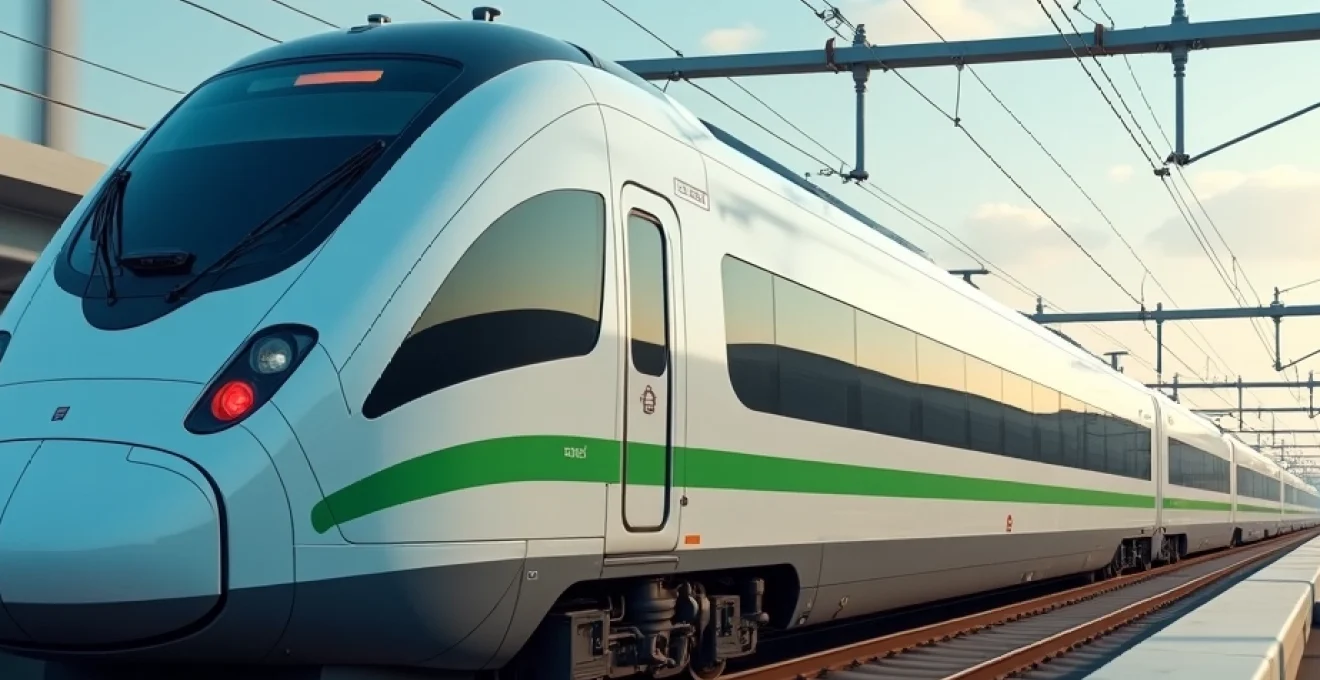
High-speed rail technology has revolutionized long-distance travel, offering a compelling blend of speed, comfort, and environmental sustainability. As countries worldwide grapple with the challenges of climate change and urbanization, high-speed rail emerges as a beacon of innovation in transportation. This marvel of modern engineering not only shrinks travel times between major cities but also significantly reduces carbon emissions compared to air and road travel. From the bullet trains of Japan to the extensive networks in China and Europe, high-speed rail continues to push the boundaries of what's possible in mass transit.
Electromagnetic suspension systems in high-speed rail
At the heart of high-speed rail technology lies the revolutionary electromagnetic suspension system. This cutting-edge technology enables trains to literally float above the tracks, drastically reducing friction and allowing for unprecedented speeds. The system works by using powerful electromagnets to levitate the train, creating a frictionless environment that traditional wheel-on-rail systems simply cannot match.
The most advanced form of this technology is found in maglev (magnetic levitation) trains. These trains use a combination of repulsive and attractive magnetic forces to achieve levitation and propulsion. The track contains a series of magnets that interact with the magnets on the train, creating a smooth and stable ride even at extreme speeds. This technology eliminates the need for wheels, axles, and transmissions, resulting in a quieter, more efficient, and lower-maintenance system.
Maglev vs. Wheel-on-Rail: comparative analysis
While both maglev and traditional wheel-on-rail systems have their merits, maglev technology offers several distinct advantages:
- Higher top speeds (up to 600 km/h for maglev vs. 350 km/h for wheel-on-rail)
- Reduced maintenance due to lack of mechanical contact
- Smoother ride quality with less vibration
- Ability to handle steeper gradients and tighter curves
However, wheel-on-rail systems remain more prevalent due to their compatibility with existing infrastructure and lower implementation costs. The choice between the two often depends on factors such as route requirements, budget constraints, and long-term operational goals.
Energy recuperation in braking systems
High-speed rail systems employ sophisticated energy recuperation techniques during braking, significantly enhancing their overall efficiency. When a train decelerates, its kinetic energy is converted into electrical energy through regenerative braking. This recaptured energy can then be fed back into the power grid or used to power onboard systems, reducing the train's overall energy consumption.
For example, some modern high-speed trains can recover up to 30% of the energy used during acceleration through regenerative braking. This not only improves energy efficiency but also reduces wear on mechanical braking systems, leading to lower maintenance costs and extended component lifespans.
Superconducting magnets in JR-Maglev SCMaglev
Japan's SCMaglev (Superconducting Maglev) system represents the pinnacle of magnetic levitation technology. This system utilizes superconducting magnets cooled to extremely low temperatures to achieve unprecedented levels of magnetic field strength and stability. The use of superconducting magnets allows for a larger gap between the train and the guideway, reducing air resistance and enabling even higher speeds.
The SCMaglev system has achieved a world record speed of 603 km/h during testing, showcasing the immense potential of this technology. While still in development for commercial use, the SCMaglev promises to revolutionize long-distance travel, potentially reducing journey times between major cities to a fraction of what they are today.
Propulsion power supply and distribution
Efficient power supply and distribution systems are crucial for the operation of high-speed rail networks. Most high-speed trains utilize electric propulsion, drawing power from overhead catenary lines or a third rail system. The power is typically supplied at high voltages (15-25 kV AC or 1.5-3 kV DC) to minimize transmission losses over long distances.
Advanced power electronics onboard the train convert this high-voltage supply into the appropriate form for the traction motors. These systems must be capable of handling rapid changes in power demand as the train accelerates, decelerates, or maintains high speeds. Some modern high-speed rail systems also incorporate energy storage devices such as batteries or supercapacitors to smooth out power demand and improve overall efficiency.
Aerodynamic design principles for rail efficiency
Aerodynamics plays a crucial role in the design and performance of high-speed trains. As train speeds increase, air resistance becomes the dominant force opposing motion, accounting for up to 80% of the total resistance at speeds above 300 km/h. To overcome this challenge, engineers employ sophisticated aerodynamic design principles to minimize drag and improve energy efficiency.
The nose of a high-speed train is perhaps its most distinctive feature, often resembling the beak of a bird or the nose of an aircraft. This elongated, streamlined shape is carefully optimized to reduce air pressure buildup at the front of the train, allowing it to slice through the air with minimal resistance. Computer simulations and wind tunnel testing are used to refine these designs, often resulting in unique and visually striking train profiles.
Aerodynamic efficiency is not just about aesthetics; it directly impacts the train's performance, energy consumption, and operating costs.
Beyond the nose, every aspect of the train's exterior is designed with aerodynamics in mind. This includes:
- Smooth, continuous surfaces to minimize turbulence
- Covered bogies and inter-car gaps to reduce air resistance
- Optimized pantograph designs for minimal drag
- Carefully shaped underbody to manage airflow beneath the train
These aerodynamic optimizations not only improve energy efficiency but also contribute to passenger comfort by reducing noise and vibration at high speeds. Additionally, they help mitigate the effects of pressure waves when trains enter tunnels, a phenomenon that can cause discomfort for passengers and potentially damage tunnel infrastructure.
Advanced propulsion technologies: linear induction motors
Linear induction motors (LIMs) represent a significant advancement in high-speed rail propulsion technology. Unlike conventional rotary motors, LIMs produce linear motion directly, eliminating the need for complex transmission systems. This technology is particularly well-suited for high-speed applications due to its ability to deliver high thrust at low speeds and maintain efficiency at high speeds.
In a LIM system, the primary part of the motor (typically containing the windings) is mounted on the train, while the secondary part (usually a conductive plate) is installed along the track. When the primary is energized, it creates a traveling magnetic field that induces currents in the secondary, resulting in a propulsive force that moves the train forward.
The advantages of LIM technology in high-speed rail applications include:
- Improved acceleration and deceleration performance
- Reduced mechanical wear due to contactless operation
- Better performance on steep gradients and in adverse weather conditions
- Lower maintenance requirements compared to traditional propulsion systems
While LIM technology is not yet widespread in conventional high-speed rail, it has found applications in urban transit systems and is a key component of some maglev train designs. As the technology continues to evolve, it may play an increasingly important role in the future of high-speed rail propulsion.
High-speed rail network infrastructure
The success of high-speed rail systems depends not only on advanced train technology but also on sophisticated network infrastructure. This infrastructure must be meticulously designed and maintained to ensure safe, efficient, and comfortable operation at high speeds. Key components of high-speed rail infrastructure include track systems, signaling, power supply, and station facilities.
Track geometry and curve radius optimization
High-speed rail tracks require precise geometry to maintain stability and passenger comfort at high speeds. This includes careful consideration of factors such as curve radii, superelevation (banking of curves), and transition curves. For example, the minimum curve radius for a train operating at 300 km/h is typically around 4,000 meters, significantly larger than that required for conventional rail.
Track alignment must be designed to minimize lateral forces on the train, which can cause discomfort for passengers and increase wear on both the track and rolling stock. Advanced computer modeling and optimization techniques are used to design track layouts that balance performance, comfort, and construction costs.
Ballastless track technology for stability
Many modern high-speed rail lines utilize ballastless track systems, also known as slab track. This technology replaces the traditional ballast bed with a concrete or asphalt base, providing several advantages for high-speed operation:
- Improved track stability and geometry retention
- Reduced maintenance requirements
- Lower structure height, allowing for smaller tunnel diameters
- Better load distribution, reducing pressure on the subgrade
While the initial construction costs of ballastless track are higher than traditional ballasted systems, the reduced maintenance requirements and longer lifespan often make it a more cost-effective solution over the long term, particularly for high-speed applications.
Noise barriers and vibration dampening techniques
Managing noise and vibration is a critical aspect of high-speed rail infrastructure design, particularly in urban and environmentally sensitive areas. Advanced noise barriers are employed along the track to minimize the impact of train operations on surrounding communities. These barriers are often designed with sound-absorbing materials and aerodynamic profiles to maximize their effectiveness.
Vibration dampening techniques are also crucial for reducing the transmission of ground-borne vibrations from high-speed trains. This can include the use of resilient materials in the track structure, such as under-sleeper pads or floating slab systems, which help isolate the track from the surrounding environment.
Signaling systems: ETCS and CTCS integration
High-speed rail operations require advanced signaling systems to ensure safe and efficient train movements at high speeds. The European Train Control System (ETCS) and the Chinese Train Control System (CTCS) are two of the most advanced signaling systems used in high-speed rail networks worldwide.
These systems utilize a combination of trackside and onboard equipment to continuously monitor train position, speed, and other critical parameters. They provide real-time information to train drivers and can automatically intervene if necessary to prevent unsafe conditions. The integration of these systems allows for increased line capacity, improved safety, and interoperability between different rail networks.
Advanced signaling systems are the backbone of safe and efficient high-speed rail operations, enabling trains to run at high frequencies while maintaining the highest levels of safety.
Environmental impact assessment of High-Speed rail
High-speed rail is often touted as an environmentally friendly alternative to air and road travel, but a comprehensive assessment of its environmental impact requires consideration of multiple factors. While high-speed trains generally produce lower emissions per passenger-kilometer than cars or planes, the construction and operation of high-speed rail infrastructure can have significant environmental implications.
One of the key environmental benefits of high-speed rail is its potential to reduce greenhouse gas emissions from the transportation sector. A study by the International Union of Railways (UIC) found that high-speed rail can emit up to 90% less carbon dioxide per passenger-kilometer than air travel on comparable routes. This reduction is primarily due to the high capacity of trains and their ability to use electricity from low-carbon sources.
However, the environmental impact of high-speed rail construction can be substantial. Large-scale infrastructure projects often require significant land use changes, potentially affecting local ecosystems and biodiversity. The production of materials like concrete and steel for tracks and structures also generates considerable emissions. A life cycle assessment approach is necessary to fully understand the net environmental impact of high-speed rail projects.
Energy efficiency is another important consideration. Modern high-speed trains incorporate numerous features to minimize energy consumption, including:
- Aerodynamic designs to reduce air resistance
- Lightweight materials to decrease train mass
- Regenerative braking systems to recover energy during deceleration
- Efficient electric propulsion systems
These features, combined with high passenger capacity, contribute to the overall energy efficiency of high-speed rail systems. For example, Japan's Shinkansen network has been reported to consume approximately one-sixth the energy per passenger-kilometer compared to air travel on similar routes.
Noise pollution is a significant environmental concern for high-speed rail operations, particularly in urban and suburban areas. Advanced noise mitigation techniques, such as sound barriers and vibration dampening systems, are essential components of modern high-speed rail infrastructure. Additionally, ongoing research into quieter train designs and track systems aims to further reduce the acoustic impact of high-speed rail.
Global high-speed rail projects and innovations
High-speed rail technology continues to evolve rapidly, with numerous projects and innovations underway around the world. These developments are pushing the boundaries of speed, efficiency, and sustainability in rail transportation.
China's Beijing-Shanghai High-Speed railway
China has emerged as a global leader in high-speed rail development, with the Beijing-Shanghai high-speed line serving as a flagship project. This 1,318-kilometer route, completed in 2011, showcases China's ability to rapidly deploy advanced rail technology on a massive scale. The line features:
- Trains capable of operating at speeds up to 350 km/h
- Advanced signaling and control systems for high-frequency operations
- Extensive use of viaducts and bridges to minimize land use impacts
- Integration with urban transit systems for seamless connectivity
The success of the Beijing-Shanghai line has spurred further high-speed rail development across China, with the country now boasting the world's largest high-speed rail network, spanning over 38,000 kilometers.
Japan's Shinkansen N700S series advancements
Japan, the pioneer of high-speed rail with its Shinkansen system, continues to innovate with the introduction of the N700S series trains. These new trains incorporate several cutting-edge features:
- Improved aerodynamics for reduced energy consumption
- Advanced active suspension systems for enhanced ride comfort
- Lithium-ion battery systems for emergency propulsion during power outages
- Modular design allowing for easier customization and maintenance
The N700S series represents Japan's ongoing commitment to refining high-speed rail technology, focusing on incremental improvements in efficiency, comfort, and safety.
European Union's Trans-European transport networks (TEN-T)
The European Union's TEN-T initiative aims to create a cohesive, high-speed rail network across Europe, linking major cities and economic centers. This ambitious project involves:
- Standardization of signaling systems through the European Rail Traffic Management System (ERTMS)
- Development of cross-border high-speed lines to improve international connectivity
- Integration of high-speed rail with other transport modes for seamless multi-modal travel
- Focus on sustainability and reducing the environmental impact of transportation
The TEN-T project faces challenges in coordinating efforts across multiple countries but has the potential to transform travel within Europe, offering a sustainable alternative to short-haul flights.
California high-speed rail: challenges and progress
The California High-Speed Rail project aims to connect San Francisco and Los Angeles with a high-speed line capable of speeds up to 350 km/h. While the project has faced numerous challenges, including cost overruns and delays, it represents a significant step towards introducing high-speed rail in the United States. Key aspects of the project include:
- Use of renewable energy sources to power the trains, aiming for zero-emission operations
- Integration with existing urban transit systems to maximize ridership and efficiency
- Implementation of advanced earthquake protection measures due to the region's seismic activity
- Potential to serve as a model for future high-
speed rail projects in the United States
Despite the challenges, the California High-Speed Rail project represents a significant step towards modernizing America's transportation infrastructure and reducing carbon emissions from the transport sector. Its success could pave the way for similar projects across the country, potentially transforming long-distance travel in the United States.
Environmental impact assessment of high-speed rail
While high-speed rail is often lauded for its environmental benefits, a comprehensive assessment requires a nuanced approach. Let's delve deeper into the environmental implications of high-speed rail systems:
Carbon footprint analysis
High-speed rail's carbon footprint can be significantly lower than that of air or road travel, especially when powered by renewable energy sources. A study by the International Union of Railways found that high-speed rail emits about 17 grams of CO2 per passenger-kilometer, compared to 153 grams for cars and 195 grams for airplanes on similar routes.
Land use and biodiversity impact
The construction of high-speed rail lines can have significant impacts on land use and biodiversity. However, when compared to the land required for equivalent highway capacity, rail often proves more efficient. For instance, a double-track high-speed rail line can carry the same number of passengers as a six-lane highway while using only a third of the land.
Energy efficiency comparisons
High-speed trains are remarkably energy-efficient when operating at capacity. For example, Japan's Shinkansen consumes about 0.33 kWh of electricity per seat-kilometer, compared to 0.48 kWh for a Boeing 777-200 aircraft. This efficiency translates to significant energy savings over the lifespan of the rail system.
Noise and vibration mitigation
While high-speed trains can generate significant noise, modern designs and infrastructure techniques have made great strides in mitigation. For instance, the use of noise barriers, vibration-dampening track beds, and aerodynamic train designs have reduced noise levels by up to 10 decibels in some cases.
The environmental impact of high-speed rail extends beyond operational emissions, encompassing construction, land use, and long-term effects on travel behavior and urban development.